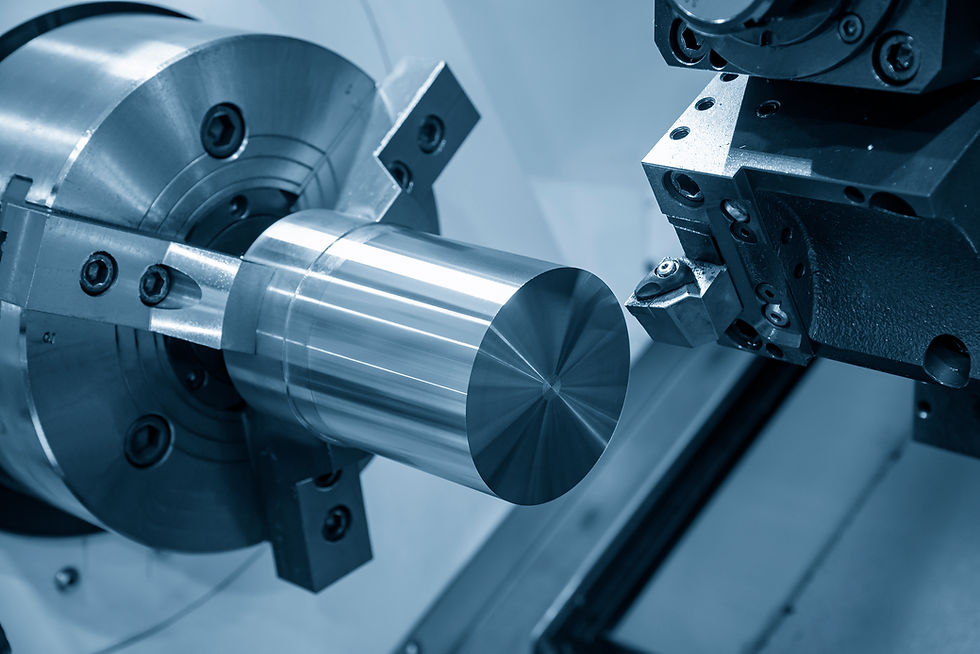
A fairly reflective surface is regularly achieved by a variety of processes, such as machining with geometrically defined cutting edges (milling, turning, planing) and machining with geometrically indeterminate cutting edges (grinding, vibratory grinding, polishing), electrochemical ablation (surface ablation), thermal ablation (e.g. laser ablation, ion beam ablation), etc. A multi-stage production process inevitably requires more resources. A multi-stage production process inevitably requires more resources, which ultimately affects the price of the end product. The problem described above can be solved economically and qualitatively by ultrasonic-assisted machining. This method has already proven its worth and shows significantly higher productivity, machining accuracy, better chip breaking properties and others compared to conventional machining. In addition, it has been successfully used for ductile ultra-precision machining of brittle-hard materials such as glass and sintered tungsten carbide by reducing the material's tendency to brittle fracture. Ultrasonic-assisted machining is an advanced manufacturing process used in metalworking and other industries to improve the efficiency and precision of the machining process. This process combines conventional cutting techniques such as milling, drilling or turning with high-frequency ultrasonic vibrations to optimise the cutting properties of workpieces.
Oscillation energy
An ultrasonic vibration generator produces high-frequency vibrations that are transmitted to the workpiece via the tool. These vibrations generate microscopic movements of the tool, which leads to improved chip formation and reduced friction.
Advantages
Ultrasonic-assisted machining offers several advantages, including a higher surface finish, longer tool life, lower cutting force, increased machining speed and the ability to machine difficult-to-machine materials such as hardened steel, ceramics and composites more efficiently.
Applications
This process is used in various industries, including the manufacture of precision parts for the aerospace, medical technology, electronics and automotive industries. Y. Song et al. used elliptical vibratory cutting to machine a cobalt-chromium alloy with a monocrystalline diamond tool. In the experiment, diamond tools with a tip radius of 1 mm, clamping and clearance angles of 10 and 15 degrees were used accordingly. Piezo actuators were used to achieve circular vibrations (with a radius of 1 µm) of the tool tip at a frequency of 15.48 kHz. Other process parameters were: Cutting speed 190 mm/min, cutting depth 10 µm and feed rate 20 µm/rev. By reducing the cutting force, work hardening was minimised, which leads to an increased dislocation density at the nano level. Tool wear, in particular edge microwear, was significantly reduced by reducing the temperature effect on the diamond tool and surface quality was positively influenced (Figure 2). A fine mirrored surface with a maximum surface roughness Rt = 25 nm was maintained up to a cutting length of about 14 metres. Figure 3 shows a possible practical approach of elliptical vibratory chipping for mirror finishing of a femoral head. [1]
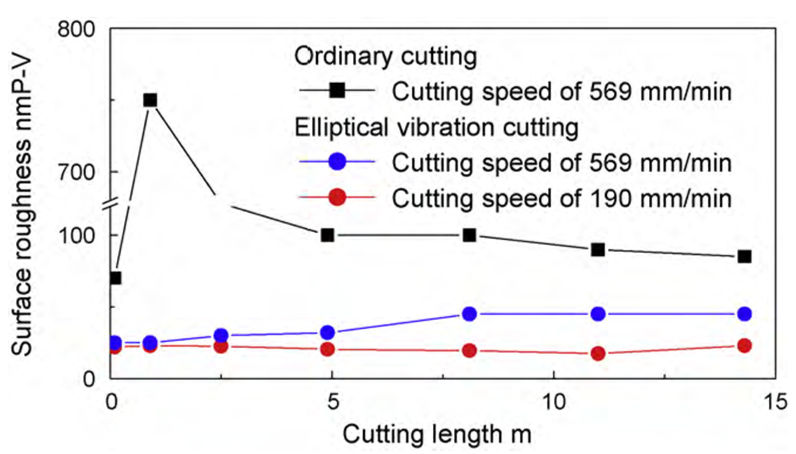
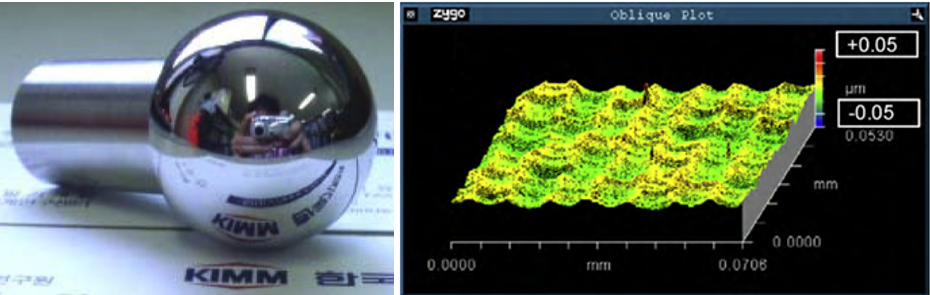
Challenges
The implementation of ultrasonic-assisted machining requires specialised equipment and expertise to set the optimal parameters for different workpieces and materials. The technology can be more expensive than conventional machining processes, but the benefits in terms of quality and efficiency can justify the cost.
Overall, ultrasonic-assisted machining enables the machining of materials with higher precision and efficiency and has found wide application in various industries. It is an advanced technique for tackling challenging machining tasks and producing high-quality workpieces.
Reference:
[1] Young Chan Song, Chun-Hong Park, Toshimichi Moriwaki “Mirror finishing of Co–Cr–Mo alloy using elliptical vibration cutting” South Korea, Japan